Thus, pricing of transmission and
distribution services has two corresponding basic elements:
- Charges for recovering the capital investment and operation and
maintenance costs for the transmission and distribution network facilities and
equipment.
- Charges for assuring system reliability and security through
so-called ancillary services and congestion management.
When an electricity industry is
organized as a vertically integrated regional monopoly, transmission and
distribution service charges have generally been integrated with generation,
general administration and other charges to form a single rate for customers
within given classes, such as residential, commercial, and industrial. This
single rate for a specific customer class is usually developed by allocating a
part of the total required revenues for the industry to this class and then
dividing the allocated revenue requirement by the forecasted annual kWh to be
sold to customers in this class.
In an unbundled, restructured
industry it is desirable to separate generation-related charges from
transmission and distribution charges to avoid potential cross-subsidies.
After restructuring, generation charges can then be determined empirically by
competitive market bidding or through bilateral contract negotiation. However,
it is often difficult to untangle the various cost components of the
transmission and distribution system, because they are associated with the
special physical characteristics of the electrical network as well as those of
the power flows inside the network, which cannot be easily isolated.
A widely used approach to reduce
the complexity of the pricing mechanism is to separate the transmission and
distribution system and their costs into various parts and then apply
computational methods that strive for an empirical balance among economic
efficiency, social equity, and computational practicality. The choice of
pricing mechanisms influences the cost of power (both the cost to generators
and the cost of energy delivered to customers), investment signals to new generation
and possibly to owners of transmission facilities, and the financial prospects
for owners of existing generation and transmission assets. The various
outcomes of different pricing mechanisms may be modeled using simulation models
that combine representations of both the behavior of market participants and
the physical transmission grid. This simulation modeling can contribute to
decisions regarding the selection of transmission pricing mechanisms.
RTOs will evaluate the effects of
differing pricing approaches for transmission services, while distribution
services and their pricing will remain the responsibility of utility
distribution companies (UDCs). Because of the large amount of electricity involved
and significant differences among customer services provided, it is usual to
delineate the costs for transmission systems in greater detail than those for
distribution system services. Major categories of transmission service charges
include:
-
System connection charges,
-
Wheeling, Access and Use charges, including
- Capital investment charges,
- Operation and maintenance charges, and
- Congestion management charges
-
Ancillary service charges, including
- Scheduling, system control and dispatch
- Energy imbalance
Operating reserves
- Spinning and supplemental reserves
- Reactive supply and voltage control
- Regulation and frequency response
- Black start capability
Determining the computational
basis for these charges is a delicate and complex exercise that has direct
impact on the interests of owners and users of the transmission system. The
establishment of these charges is typically subject to regulation, almost
always by the FERC since the transmission network involves generators and loads
in several states (the main exception is the Texas grid, which is not
synchronized to either the Eastern or Western Interconnect, and is regulated by
the Public Utilities Commission of Texas. The regulatory process involves
balancing economic efficiency, social equity, the need to cover the financial
and physical costs of the network, and administrative practicality. Modeling
for RTOs must show both the flow of payments associated with the various
charges, and address the efficiency and equity issues of each.
System
Connection Charges
Among these charges, system
connection charges are probably the most straightforward and can often be
determined by using an average charge for the connecting equipment and
assigning a distance-related charge for the transmission line required to
connect the new customers.
Wheeling, Access and Other Usage Fees
Charges related to use of the
transmission grid itself involve wheeling charges assessed to transactions
originating and/or terminating outside the RTO or ISO, other access charges
assessed to all transactions using the transmission network, and fees assessed
to users of constrained transmission facilities (known as congestion charges).
In the pre-ISO/RTO environment,
the dominant methodology for managing transactions involving generators in one
pricing region and customers in another is the so-called contract path
approach. This involves the assembly of transmission reservations on a
sequence of connected transmission paths leading from the generator (source) to
load customer (sink). A typical contract path might cross the transmission
systems of several transmission owners, each of which might require payment of
a wheeling or access charge in order to schedule the transaction. While this
approach is administratively convenient, it corresponds poorly to the physical
reality of the transmission grid. In general, a balanced injection of power at
one point and removal of power at another point changes flows on all paths of a
network, not just on the contracted set of linking paths; the flows off the contract
path are called loop flow, and are an unpriced use of network
assets, which in addition complicates the real-time management of the
interconnected grid. In addition, as will be discussed below, usage fees
unlinked to transmission constraints are inefficient, and the pancaking
of such fees discourages efficient inter-regional trading of power. These two
sets of problems—loop flow and pancaking—are primary motivations for Order
2000, and modeling these issues is likely to be important to policy filings in
response to the order. In any event, contract-path approaches may continue to
be used to price at least some power transactions, at least until a full system
of RTOs is in place, with arrangements to avoid pancaking.
For capital investment and operation
& maintenance charges, it is generally difficult to prescribe a precisely
fair allocation of costs among either the customers or the generators beyond
demand (MW) related and energy (kWh) related components. Thus, for simplicity
it is common to apply a postage-stamp approach to recover revenue
requirements equally from all customers regardless of transmission distances.
An efficiency concern arises when the postage-stamp charge is not related to
the opportunity costs of the transactions to which it applies. Charging a fee
for the use of transmission that is operating at below capacity discourages use
of the transmission, and favors generation on the receiving or energy-deficit
side of the transmission path, relative to generation on the sending or energy-surplus
side of the path. Congestion pricing, using either the
difference in locational marginal prices between bus pairs (as is used, for
example, in PJM), or the difference between prices in fixed zones (as in the
California ISO), is more efficient. However, the revenues from congestion
pricing (including revenues derived from the sale of congestion-based financial
instruments such as Firm, Fixed, and /or Financial Transmission Rights [FTRs])
may be insufficient to meet the financial and physical maintenance requirements
of many transmission assets; the non-congestion transmission-access and
wheeling charges are a mechanism to finance these stranded assets.
Related sets of charges are those
based on the distance. These include MW-mile access charges and rules for
accounting for network losses.
MW-mile charging for bilateral
contracts, based on the distance between source and sink, have some appeal as
they seem to tie charging for the grid to the physical grid resources used.
However, it has been argued that MW-mile charging for grid access amounts to
nothing more than “smooth pancaking”, inefficiently discouraging use of
below-capacity transmission facilities.
Charges to account for
transmission losses, on the other hand, can reflect an economic cost to
distance. A standard methodology for computing losses uses a load-flow model
to estimate the change in total losses that results from a simultaneous 1MW
increase in generation by the generator and a 1 MW increase in total loads.
Various approaches are used to assign or otherwise account for these losses.
In the California system, losses are computed ex-post, and a generator must buy
balancing energy to make up for its losses (but it can avoid these charges by
increasing its generation by a sufficient amount). Alternatively, in a
centrally controlled system (such as PJM), losses may be handled implicitly in
the optimization process, with generation that contributes relatively heavily
to losses receiving a lower locational marginal price (LMP) than does
generation that tends to reduce losses. Given accurate models of the physical
grid, both approaches produce price signals to generation that accurately
reflects the individual plant’s transmission losses due to its location in the
network. Furthermore, the loss pricing produced by either model can be
reproduced in a simulation model, so long as the simulation model also
accurately represents the transmission grid.
To the extent that transmission
rates (in particular wheeling and access charges) are not based on
economic-cost principles (as are both congestion pricing and transmission-loss
accounting), but instead are justified as cost-recovery arrangements (typically
related to filed revenue requirements), rate designers (including in particular
RTO managers) may adopt performance-based ratemaking principles,
which are innovative regulatory schemes designed to accommodate cost-recovery
requirements while delivering efficiency-enhancing incentives to the regulated
utility. An example of such an arrangement is a fixed stranded-asset charge,
which is not subject to retroactive adjustment for additional profits from the
market. To set levels for such rates requires careful analysis of market
behavior under the rates, and analysis which is equally necessary for utilities
considering operation under the proposed arrangements. Even where innovative
rates are not included, due diligence would require the evaluation of
prospective rates to ensure that RTO/ITC business strategies do not hinder
opportunities for future innovative rates or products.
Some key challenges in evaluating
performance-based rates in particular include:
- defining appropriate performance benchmarks, perhaps based on congestion costs (several measures are possible) and market efficiency,
- projecting and allocating among market participants the resulting savings or penalties for performing better or worse than benchmarks, and
- projecting subsequent market responses, including bidding and prices (e.g., for energy, A/S, balancing energy, transmission rights), as well
as future investment decisions.
As discussed above, the pricing
system established by each RTO for use of the power grid will typically involve
some combination of fixed grid-usage charges and congestion-management charges
for the use of constrained paths. These charges will have a direct impact on
the prices that generators receive at different locations. Thus, they can
influence the pattern of energy dispatch and the locational incentives received
by individual generators. In a full multiple-market analysis, transmission
charge rules and different tariff arrangements, short-run energy dispatch and
costs, longer-term investments in generation, and transmission flows and
revenues can be evaluated simultaneously to estimate effects on power flows,
revenues and profits over time.
When congestion arises along a
flow path, the costs of operating the system are likely to rise and operating
limits are more likely to be exceeded. Since one of the RTO’s primary
functions is to ensure that physical dispatch remains consistent with loads and
the physical capabilities of the grid, congestion management services have been
developed to deal with load curtailment, system rebalancing, congestion
payments and other related issues.
The costs of redispatch to
relieve congestion and ensure more reliable grid operations can affect revenues
earned by transmission owners and determine their incentives for maintenance
and expansion of the transmission grid. One way to deal with congestion is to
create a market for Firm Transmission Rights (“FTR). FTRs are supposed to
encourage the efficient use of transmission assets through an economic market.
For example, when a constraint emerges on a particular flow path, the
constraint would be measured via the market price for FTRs. The changing market
price would provide an economic incentive for Scheduling Coordinators to adjust
their schedules to free up some transmission capacity, in order to allow higher
value transactions to take place. Hence, the presence of FTR and recallable
and non-firm transmission rights can provide economic incentives for market participants
and permit the RTO to actively manage congestion. Nevertheless, because
market participants have differing incentives, devising workable FTRs is a
process that many RTOs have yet to implement.
Congestion management charges are
the most complex, because they depend on not only a more complicated protocol
of power wheeling, but also on whether there is an open market for electric
generation. The development of these charges varies from simple postage-stamp
cost allocation, such as in the UK, to a market-based price determination, as
in California.
To identify and manage congested
transmission lines or to implement FTRs, a model that integrates market
behavior with Optimal AC Power Flow can be applied to project both the levels
and costs of redispatch to relieve congestion through time, to compare the
costs and benefits of additional transmission or generation projects that could
relieve the congestion, and to estimate congestion revenues.
While power marketers typically
engage in nominal point-to-point transactions by making the more-or-less
implicit assumption of a single path between injection and take-out points, the
RTO must model the full impact of transactions on the grid. This typically
involves every node combination in the network. Even if the RTOs formal
congestion management rules (such as pricing of congested transmission
as if the grid were a small number of radially-connected hubs) are simplified
for the convenience of grid users, the grid manager must respond to the physical
properties of the full grid. Thus, market modeling that incorporates a full
grid model (such as an OPF) can identify flows across all nodal pairs and
compare flows under alternate arrangements.
By eliminating pancaked tariffs
and creating larger geographic areas under RTO control, the FERC hopes to
reduce loop flows. By projecting flows on designated transmission lines across
particular interfaces, the effects of alternative tariff designs, differing
geographic boundaries and expanded RTO membership can be assessed.
The provision of adequate
contingency reserves (such as spinning and non-spinning ten-minute reserves),
as well as the facilities for the maintenance of instantaneous balance between
loads and resources, is a critical task of the RTO. In Order No. 888, the FERC
designated six Ancillary Services: 1) Scheduling, system control and dispatch
service; 2) Reactive supply and voltage control from generation sources
service; 3) Regulation and frequency response service; 4) Energy imbalance
service; 5) Operating reserve – spinning reserve service; and 6) Operating
reserve – supplemental reserve service. Black start capability is sometimes
considered an ancillary service, but it is procured separately from the others
and is called upon infrequently compared to the other services.
Within these categories different
“reliability products” may be provided. These services are distinguished
primarily by the responsiveness of the generators supplying the requested
service.
Since these services require
capacity, they add to the cost of delivering power. Some arrangements may
cause relatively low-cost generation to remain outside the energy dispatch,
causing the dispatch of higher-cost generation. Effective modeling of the
electric energy market requires that the A/S procurement and charging rules be
incorporated into the model. In markets with RTO-managed dispatch of A/S, this
requires modeling of the bidding behavior of participating generators and
eliminating arbitrage opportunities among the multiple A/S markets.
Recovery of ancillary services
charges are complex, because they involve not only the transmission system, but
also the special characteristics of the generators and the loads they serve.
Determination of these charges generally starts with a set of pre-specified
system standards for the services, such as the amount of each type of reserve
required. Setting charges for these services also depends on the specific
protocols in each region. In some regions, generators can self-provide
ancillary services, so the costs will be internalized. This is the case for
the PJM Interconnection. In other areas, the system operator will charge the
generator based on a set of rules and postage-stamp type rates. This is the
case in Australia, the UK, and Japan. In still other regions, the system
operator will purchase services from the ancillary service market and charge
market prices. This is the case in California.
Open Access Same-time Information
Systems (OASIS) is a fundamental service of RTOs and other providers of
FERC-jurisdictional transmission systems. These systems provide timely
information about the total and available transfer capability of the
transmission grid. They are used by power marketers and others to develop
transactions linking generation with loads. In the process of modeling the
transmission grid, an integrated power-market model requires monthly total
transfer capability (TTC) information, and will calculate the hourly available
transfer capability (ATC) as an output.
There are several approaches to
the modeling of market power and other competitive aspects of power markets.[3]
First, the traditional reliance on market-power indices (such as the well-known
Hirschman-Herfindahl Index, or HHI) can be quantified for any dispatch
situation, provided that the market model is capable of aggregation to
owners/operators. Second, a market model that simulates both prices and costs
at specific locations can support the more direct measurement of the effects of
market power through computation of markup-related indices such as the Lerner
Index, or through simulations of welfare measures such as consumer or producer
surplus. In addition, the market and power flow effects of measures such as
price caps or plant divestiture can be analyzed to help satisfy FERC’s required
market monitoring function.
Transmission planning is
necessarily coordinated with generation expansion and load growth. In a
market-mediated transmission expansion analysis, simulation of energy
deliveries over time allows estimation of congestion rents and the value of
FTRs over time. This quantification can, in combination with projections of
grid access charges, produce projected revenue streams sufficient to justify
and finance new transmission expansions. In a more conventional
transmission-planning context, an integrated generation/transmission market
model can predict the location, frequency, timing and probability of future
transmission insufficiency, thus contributing to the transmission planning
exercise. An example of this process for assessing reliability is discussed
later in this section.
Interregional coordination
involves the development of protocols across different control regions to allow
the effective operation of the entire interconnected grid. The effects of
protocols between regions can be modeled just like the other elements of the
transmission and dispatch tariff, including a variety of restrictions on interregional
dispatch and reserve-provision practices.
Each of the FERC’s required RTO
functions is likely to affect the others. The UPLAN Network Power Model has
been designed to provide the necessary integration, technical accuracy and
flexibility to analyze these effects. Table 1 illustrates how UPLAN addresses
RTO functions:
FERC’s RTO Functions
|
UPLAN Treatment of Mandatory Functions
|
Tariff
design & administration
|
Directly
addressed via evaluation of alternative transmission tariffs and resulting
contract flows, producing projected revenues and power transaction
volumes.
|
Transmission
congestion management
|
Directly
determined by optimal AC power flow analysis and UPLAN redispatch costs to
relieve congestion.
|
Parallel
path flow (reduced loop flow)
|
Directly
identified from calculated flows on specified paths and by simulation of
measures affecting power flows.
|
Ancillary
services
|
Explicitly
simulated by applying market design protocols: e.g., UPLAN treats CA, New
England & PJM rules in its bidding and ISO dispatch functions for
regulation/AGC, spin and non-spin reserves, replacement and real-time
imbalance energy.
|
Total
Transmission Capability (TTC) and Available Transmission Capability (ATC)
|
TTC
is input into the database for each line and interface and may vary by month.
ATC is simulated hourly by the AC power flow calculation.
|
Market
monitoring
|
Direct
calculation of multiple measures of generator market power, as well as impact
measures of bidding behavior, capacity withdrawal, and the effects of price
caps.
|
Transmission
planning and expansion
|
Analyzed
via alternative scenarios and built-in new entrant model for generation
expansion, additions and retirements. Develops measures of transmission
system adequacy.
|
Interregional
coordination
|
UPLAN
models each NERC region, as well as the Eastern, Midwestern and Southeastern
Interconnected Systems, WSCC & ERCOT, plus flexibly defined RTO regions
embedded within each regional grid.
|
Several examples of UPLAN
applications to evaluate RTO reliability-related functions are described in the
following sections.
III. The
Reliability Problem: Modeling Generation and Transmission System Adequacy
One of the RTO’s main roles is to
maintain the backbone electric transmission grid, a set of tasks that involves
coordinating, constructing, maintaining, and/or owning transmission facilities
sufficient to support the efficient operation of the region’s generating
facilities. Traditionally reliability has been measured using a static index
of Loss of Load Probability (LOLP), which takes into account only generation
outages but assumes no uncertainties in demand. LOLP is a long-term measure
often used as a criterion for generation expansion. However, in the
deregulated market, merchant plant additions are based on economic criteria.
Hence, capacity additions cannot be dictated by solely by reliability measures,
such as LOLP or reserve margin.
A number of indices may be
calculated to measure reliability on a dynamic basis. For example, the average
unserved energy at each demand node measures the demand that would be
interrupted due to shortages, transmission constraints or excessive loads. The
standard deviation of the unserved energy gives a measure of the volatility of these
occurrences. Other measures include the frequency of load interruptions, as
well as the variability and the standard deviation of load interruptions.
These numbers can be compared for cases with and before particular merchant
plant additions, transmission reinforcements or transmission capacity
additions.
In the context of the traditional
integrated utility, the transmission planning exercise is a straightforward but
computationally complicated exercise involving the selection of transmission
and generation expansion plans that minimize the total present-value cost of
delivering uninterrupted power to the utility’s customers. At every point on
the grid in every hour (and in every reasonable contingency) there needs to be
sufficient generating capacity to meet loads. However, when the transmission
grid is operated at capacity, there will be some incremental loads that can
only be served by increasing the output of local generation. In that
circumstance an integrated planning process can compare the cost of delivering
new generation to the cost of reinforcing the transmission grid to achieve an
acceptable level of reliability.
Under a market-driven
restructuring, the generation capacity decision and the transmission capacity
decision are separate business decisions. Yet, they remain inter-dependent.
The potential investor in additional generation capacity is interested in the
future energy prices at a prospective location, which are likely to be
influenced by the future state of the transmission grid. In turn, the need for
transmission and the profit opportunities for transmission reinforcements are
determined in large part by future generation expansion. In short, this is
another multi-product example, where the two reliability-delivering products
are generation capacity and transmission capacity. Here again, the challenge
is to make decisions that take account of concurrent decisions by other market
participants.
Hence, reliability-capacity
decisions are susceptible to analysis with multi-market simulation models. In
the following example we present such an analysis for the Western System
Coordinating Council. This represents an actual simulation of how an ISO or a
RTO may carry out planning function to maintain the transmission and generation
adequacy requirement of the system. The base year of the study is 2003 and
adequacy study is conducted for the future years 2004 and 2005.
UPLAN Analysis of Generation
Adequacy
We analyzed generation and
transmission adequacy planning using UPLAN model for WSCC, with load growth
projected over 10 year period from 2004 to 2013 , The model represents all the
transmission line of 230 kV and up, approximately 800 thermal and hydroelectric
generators and all the major transmission interfaces listed in the WSCC Path
Rating Catalogue[4]
For clarity, this presentation is limited major interfaces in California and
zone effecting imports to and from California. And results are provided for the
year 2005 only.. The modeling effort consists of simulation of the system before
any generating unit addition in the future years, followed by an optimal
expansion of generation and transmission for the future and finally, an
adequacy analysis after the plant additions.
Table 2: Generation Expansion Plan for the Years 2004
and 2005 for the WSCC
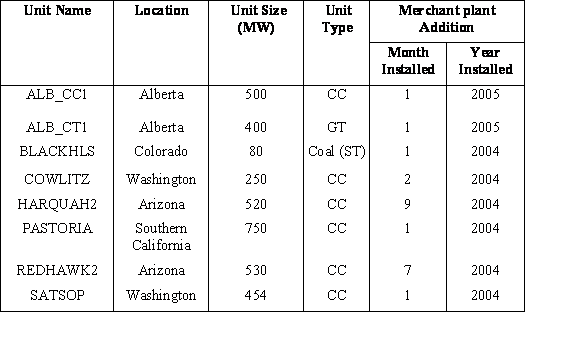
Table 2 lists the new plant
added to the system in 2003 and 2004 by UPLAN Optimal merchant Plant model for
which the net present value of the plants is positive, given expected load
growth, fuel prices and other fundamental economic variables. The. A variety
of reliability analyses were performed, using Monte-Carlo techniques to
simulate both market and physical events, such as local variations in loads,
and forced outages of generation. One can compute, for example, the probability
and expected duration of loss of load events, or the expected annual duration
of transmission congestion. We undertake fifty simulations of the system both
before and after the new plant addition.
A number of indices may be calculated to measure
reliability on a dynamic basis. For example, the average unserved energy at
each demand node measures the demand that would be interrupted due to
shortages, transmission constraints or excessive loads. The standard deviation
of the unserved energy gives a measure of the volatility of these occurrences.
Other measures include the frequency of load interruptions, as well as the
variability and the standard deviation of load interruptions. These numbers can
be compared for cases with and before and after the merchant plant additions,
transmission reinforcements or transmission capacity additions. These
occurrences are of vital interest to owners and planners of both generation and
transmission assets. Table 3 describes the nodal unserved energy reports for
2005, for both cases, showing selected nodes in WSCC. These reports suggest
that the projected unserved energy at all the nodes, on an average, is not only
high before the new units are added to the system but also frequent as compared
to the case after new unit addition. Duration curves of the average unserved
energy in WSCC given in the figure 1 indicate that both the magnitude and the
duration of the unserved energy are substantially higher before the plant
additions.
Table 3. Unserved Energy Report for WSCC, 2005
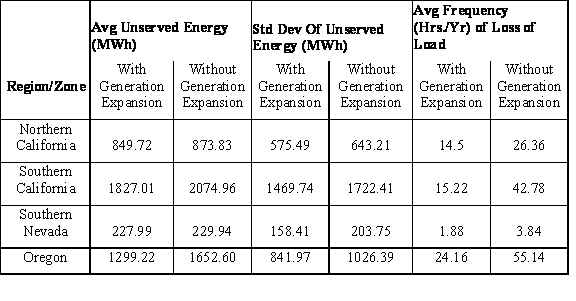
Given the mean and variance of the distribution of
average unserved energy at any node, we can calculate the additional economic
capacity required in order to minimize the unserved energy at that node.[5]
From Table 3 we can see that after all the units that are economically viable
are added to the system there are 850 MWh (Standard deviation 575)
unserved energy in Northern California. From the simulated cumulative
distribution, (from the frequency and the magnitude of unserved energy) we can
see that an addition of approximately 127 MW of uneconomical (Reliability Must
Run) capacity at the AIRPORT bus will reduce the unserved energy 40 MWh per
year. The loss incurred in this investment should be borne by the RTO as a cost
of reliability.
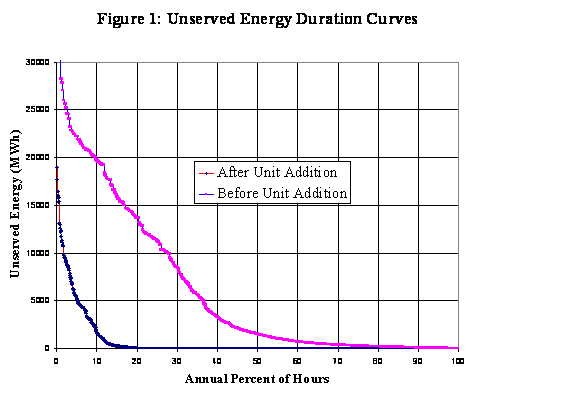
Table 4 shows an example of a transmission adequacy
report, showing both duration and marginal and total annual costs and duration
of congestion costs on selected transmission interfaces in California, given
the expected generation and loads for 2005. Figure 2 presents the
chronological flows through COI (California-Oregon Interface), Path 15,
PGE_SCE, and WOR (West of River) interfaces. The flow duration curves are
particularly helpful in analyzing the impact of an interface capacity on the
number of hours the flow across the interface is constrained and the additional
cost incurred for meeting the demand from higher cost generation. For example,
consider flow duration in case of PGE_SCE. If the interface capacity for
central to southern California is 1,000 MW, the interface will be congested for
2803 (32%) and 3679 (42%) hours before and after new capacity additions,
respectively. Also in case of south to north Path 15 flow which is constrained
we see no significant difference in the duration curves for both cases as all
of the units added are in the expansion plan are outside California, and they
do not have significant impact on the interface within California.
Figure 2: Flow
Duration Curves With and Without Generation Expansion for WSCC, 2005
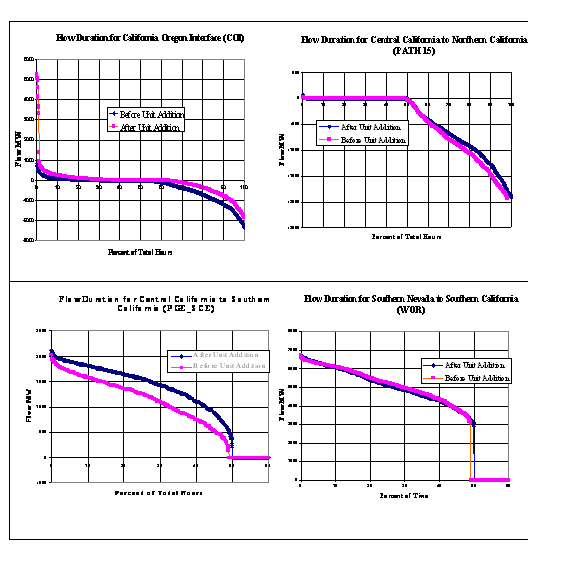
For operational planning one can obtain the probability
of congestion during a particular hour on the main interconnections, as well as
the expected congestion prices on those paths and the expected congestion cost.
The congestion costs shown here represent the differences between locational
prices on either side of the congested interface in those hours in which use of
the interface is constrained, minus relevant grid-access or wheeling charges.
It is, therefore, the marginal value of additional generation. However, if
additional transmission capacity is added the congestion will disappear and
owners of the new lines have to recover their investment through appropriate
transmission charges. It can be observed that the addition of the generating
plants, as in case (a), adds into the congestion cost on the interfaces. For
example, on an average the COI interface is congested during 183 hours in case
of generation expansion, whereas in case of no expansion, the number of
congested hours is restricted to approximately forty-nine. However, as shown in
the Figure 3, the system-side congestion costs are lower at these interfaces in
case of generation expansion than otherwise. It is important to distinguish
between the level of congestion at the interface and the system-wide congestion
due to congestion at that particular interface. We can infer that expanding
generation capacity within California will not only reduce the level and the
instances of unserved energy, but also reduce the level of average and total
congestion cost in the WSCC region.
Table 4: Projected Transmission Adequacy: Summary of
Congestion Cost at Selected Interfaces for the Year 2005 for the WSCC
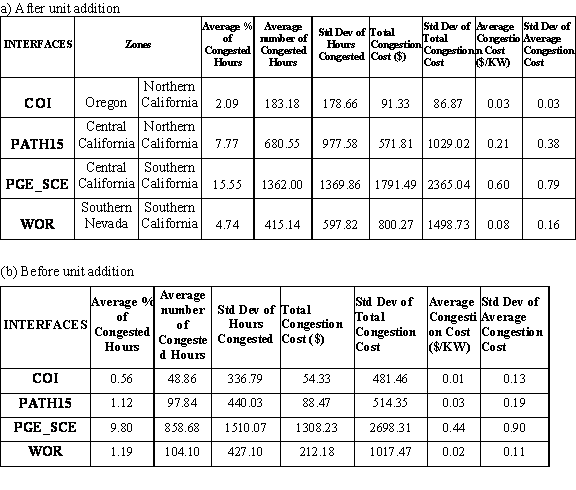
Figure 3:
Duration Curves of the Projected Congestion Cost With and Without Generation
Expansion for WSCC, 2005
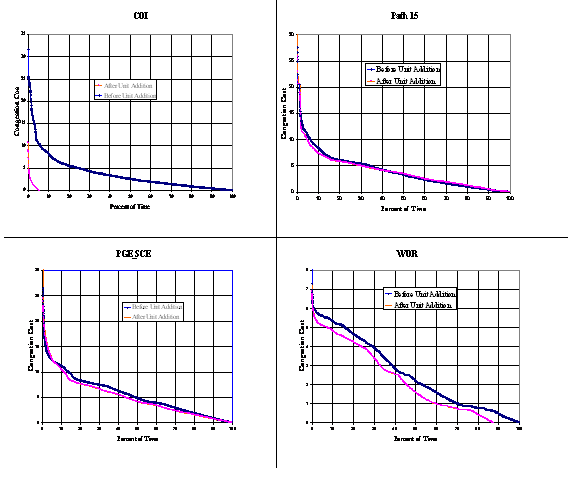
Mitigating Generation and
Transmission Inadequacy
The evaluations of future
generation inadequacy, and of present and future transmission congestion, are
components of the RTO’s critical mission of overseeing transmission grid
development and operation to allow reliable and efficient grid operations. At
least three of the RTO functions identified in FERC Order 2000 (and reviewed in
Table 1) are directly involved. First, the RTO’s performance of its tariff
design and administration function determines, in part, the ability of merchant
generation to respond to growth in loads, in the context of the ongoing
evolution of the physical transmission grid. Second, impending shortages of
generation, relative to load, produce episodes when market power may arise,
which may (depending on the regulatory environment) produce dramatic price
spikes, possible inefficiencies in grid operations and generation dispatch, and
a visible role for the RTO’s market monitors. Third, the transmission planning
and expansion function is very closely tied to analyses of future growth in
loads and resources, while (depending on the tariff design) financing of grid
expansions may be directly related to expected congestion and congestion
revenues.
As the direct manager of the
transmission grid, after primary responsibility for planning for grid
expansion, the RTO must consider both the needs for grid expansions, and the
impacts of financing such grid expansions on long-term growth of generating
resources. The congestion costs identified in tables 4 and figure 3 are a
signal for grid expansion. However, expansions that eliminate such rents would
eliminate as well the market-based means of paying for such expansions.
Reinforcing the grid to the point where congestion is eliminated would require
the imposition of usage fees on all generation and load, with no locational
price signal. However, it may be that some grid reinforcement may eliminate,
at lower cost, the need for the installation of site-specific generation, allowing
instead the construction of a few larger and more efficient plants.
Our generation adequacy analysis
indicates that expanding generation capacity can reduce the level of unserved
energy, particularly within California. The challenge for the reliability manager—ISO,
RTO, or even state regulator—is as complex as the challenge facing the investor
in new generation, or as that facing the manager, in complex and interrelated
markets, of existing generation: it is to evaluate the effectiveness of his
decisions in achieving his goals, after taking into account the actions of
competitors and of other participants in the new energy marketplace. It is the
problem of evaluating investment equilibrium over time. Rational-expectations
equilibrium pricing models, such as the UPLAN model, are effective tools to
assist the resolution of these tools, to enable the efficient management of the
power grid in a market-driven restructured industry.